Biofabrication of metastatic tumor-on-a-chip models for precision oncology
Cancer and tumors are the deadliest disease causing the death of the largest number of people. Sometimes, cancer spread from one organ and site of the body to the other sites and organs. The spread of cancer is very dangerous occurring in the later stage that causes the definite death of people. Most of the anticancer therapies fail to cure the cancer patients in this last stage.
Metastasis and secondary tumors originating from the primary tumor cause more than 90% of cancer-associated deaths (Nieto et al., 2022). A lot of researches have been conducted to understand the mechanisms of metastasis. However, mechanisms related to metastasis are not yet very clear due to its very complex process. But they have made significant progress to understand what they should do further research, in vivo and in vitro studies and clinical trials. Further studies can help understand the mechanisms of metastasis and tumor microenvironment to prevent the spread of cancer and high mortality rate resulting from it.
The complex biological processes of metastasis involve the invasion of the surrounding stroma to the circulation through the bloodstream and the arrival to and invasion of the secondary tissue (Nieto et al., 2022). This complex process was conceptualized into two phases: (i) physical translocation of the primary tumor to distant tissue and (ii) colonization and development of the secondary tumor. Cancer stem cells (CSCs) and vessel formation move from the original tumor to distant tissues through the circulatory system (Nieto et al., 2022). After that they invade, establish, and develop secondary tumor there. The complexity of the tumor microenvironment needs to be understood and accurately included in the models of metastasis.
Nieto et al. (2022) made a review to describe the advancement in the development, study, and use of tumor-on-a-chip models, focusing on the metastatic process. They reported that advanced in vitro models of cancer mimicking tumor microenvironment and simulating metastasis should be developed to understand the associated unknown mechanisms. Their review described the biofabrication of tumor-on-chips to produce the most sophisticated and optimized models for recapitulating the tumor process. Three-dimensional bioprinting technology can be combined with the novel tumor/metastasis/multiorgan-on-a-chip systems to generate models which can be used for both basic cancer research and clinical trials.
Reference
Nieto D, Jiménez G, Moroni L, López-Ruiz E, Gálvez-Martín P, Marchal JA. Biofabrication approaches and regulatory framework of metastatic tumor-on-a-chip models for precision oncology. Med Res Rev. 2022 Sep;42(5):1978-2001.
How do cancers and tumours evade the immunity? Current therapeutic options and future research
Cancer is one of the deadliest diseases causing very high death toll every day in the world. Frequently, cancer has a poor prognosis. Multiple therapeutic strategies and treatment options have been developed for various cancers and tumours. The chemotherapy, radiotherapy, targeted therapy, and immunotherapy are applied over the past several decades. However, resistance to these therapies is a major issue that impedes the clinical outcomes and effectiveness of cancer treatments and impacts patient survival. The patients with early stage positively respond to the treatments, but they poorly respond and show dire clinical outcomes at a later stage (Wu et al., 2021 and references therein).
Tumours, Structure and Immune System
The tumours produce tumour antigens that can enable the immune system to differentiate tumour cells from normal cells. Antigens trigger the immune response and activate the T cell response that can provide defense against tumourigenesis. But tumour cells develop some mechanisms to evade or avoid host immunity for their growth and survival (Spurrell and Lockley, 2014).
Tumours grow within a complex environment of structures, cells, and chemical signals ranging from epithelial cells, stroma, blood, and lymphatic vasculature, immune cells, cytokines, and chemokines. The structure of a typical tumour consists of the tumour core, the invasive margin, and the surrounding stromal and lymphoid components. Some components within the immune milieu are beneficial, while some components are deleterious to the patient. There are different immune cells identified in different locations within a tumour. The clinical outcome is presumed to be affected due to the variation in density and distribution of immune cells within tumours. The anti-tumour immunity can be increased by the down-regulation of immune checkpoint proteins. The antibodies are increasingly used to up-regulate host anti-tumour immunity with some durable results seen in early trials so far (Spurrell and Lockley, 2014).
What is Tumour Microenvironment?
The tumour microenvironment (TME) is the extracellular environment that contains tumours cells, carcinogenetic cells, cancer-associated fibroblast (CAFs), immune cells, the vasculature system, and the extracellular matrix (ECM) including secreted cytokine, chemokine, metabolites, and exosomes. The genetic and epigenetic alterations in tumour cells provide them active resistance capacity. Besides this, the tumour microenvironment (TME) plays a key role in tumorigenesis, metastasis, progression, and adaptive resistance to cancer treatment (Wu et al., 2021 and references therein).
Adaptive Mechanisms of Cancer/Tumour Resistance
Wu et al. (2021) indicated that the adaptive mechanisms of tumour resistance are intimately related to tumour microenvironment. However, adaptive mechanisms driven by the TME are not yet clearly understood and need detailed studies to fully elucidate the mechanisms of tumour therapeutic resistance. But there are some evidences that many clinical treatments targeting the TME have been successful. Cancer cells escape from the cytotoxicity of tumour therapies through the following intrinsic mechanisms, e.g., decreased drug accumulation, altered drug metabolism, mutated, or altered drug target and enhanced DNA repair capability, as well as inactivated cell death signalling. Tumour cell heterogeneity is one of the factors that creates various resistance responses for multiple therapies. Sometimes, tumours progression continues, even though they face external pressure from various therapies. Therefore, new theories have proposed that tumour progression is a dynamic and complicated process that tightly interacts with the surrounding environment (Wu et al., 2021 and references therein).
The non-malignant cells in the TME actively boost carcinogenesis by promoting excessive tumour initiation, malignant progression, metastasis, and therapeutic resistance. The transformed cancer cells contribute extensively to tumour development and resistance by interaction with stromal cells in the TME. Some preclinical studies indicate that the TME is a potential therapeutic target (Jin and Jin, 2020). For instance, multiple strategies of combined therapy related to the TME have shown interesting potential (Wu et al., 2021 and references therein).
Tumours and Immunity Evasion
The development of tumours despite host immunity indicates that tumour cells develop immune avoidance. Tumours develop immunity resistance through several mechanisms (Wu et al., 2021 and references therein):
- Some tumours have been demonstrated to lose expression of MHC molecules making them unable to present tumour antigens, thus evading T cell recognition.
- Some tumours secrete immunosuppressive cytokines, e.g., IL-10.
- Some tumours generate physical barriers, e.g., collagen and fibrin, thus making them invisible to the immune system.
- Tumours can also evade the immune response by up-regulating inhibitory molecules and inducing a form of self-tolerance.
- An immune response can be produced by T cells depending on co-stimulatory signals. However, inhibition of co-stimulatory signals creates immune tolerance. Cytotoxic T-lymphocyte associated antigen 4 (CTLA4) and programmed cell death protein 1(PD1) are both inhibitory receptors involved in down-regulation of immune responses.
Tumour Immunology, Antibody, and Clinical Outcome
The level of immune cell infiltration correlates with prognosis in many cancers. Different populations of cells within the immune infiltrate affect prognosis in different ways. Monoclonal antibodies can target different types of tumour antigens for both solid tumours and haematological malignancies. Antibodies can kill tumour cell through several mechanisms (Wu et al., 2021 and references therein).
- Direct action of the antibody: the binding of antibody to the cell causes receptor blockade that inhibits the downstream signalling pathways within the cell, ultimately leading to apoptosis.
- Immune-mediated mechanisms include antibody-dependent cellular cytotoxicity (ADCC) and complement-dependent cytotoxicity (CDC).
- The inhibition of immune checkpoint proteins by targeted antibodies, e.g., anti-CTLA4 and anti-PD1 can do the immune modulation of T cell function.
- Conjugation of a radioisotope to antibody is used as a therapeutic in lymphoma.
- Many clinical trials combining anti-PD (programmed cell death) therapy with other antitumour drugs produced no synergistic or additive effect. Therefore, Kim et al. (2022) emphasized that defining adaptive immune resistance mechanisms at the tumour site should be a key focus to direct future drug development as well as practical approaches to improve current cancer therapy.
What is Immunotherapy
Immunotherapy is one kind of cancer treatment strategies that boosts the patient’s own immune system to eliminate cancer cells in solid tumours (Xu et al., 2022). More than 70 FDA-approved immunotherapy drugs were available up to now at April 2022. A plenty of immunotherapy-related clinical trials have been registered around the world for more than 50 types of cancers. Immunotherapy mainly includes cancer vaccines therapy, oncolytic virus therapy, dendritic cell (DC) therapy, adoptive cell therapy, antibody–drug conjugates (ADCs), and immune checkpoint inhibitors (ICIs). Out of these, ICIs, which use antibodies to programmed cell death protein 1 (PD-1) and programmed death ligand 1 (PD-L1) showed the most success (Xu et al., 2022). Although new technologies single-cell multi-omics may solve many problems, cancer immunotherapy still faces many challenges.
What is Tumour Immune Microenvironment?
Solid tumour tissue consists of highly heterogeneous cancer cells (Xu et al., 2022). The different upstream mutations and the tumour microenvironment (TME) form the variable compositions and evolutionary states of these cancel cells. The composition of TME controls the tumour–host interaction. Tumour immune microenvironment (TIME), compositing different cell groups of the immune system and their interactions in the TME niche significantly control the processes of carcinogenesis, cancer progression, and responses to the treatments (Xu et al., 2022).
What is Systemic Tumour Immune Environment (STIE)?
Systemic tumour immune environment (STIE) contains the immune modulating molecules and immune cells and mainly governed by the circulating blood and lymphatic vessels (Xu et al., 2022). It has significant role in communication between the primary tumour site to the distant organs and the host immune organs such as bone marrow and lymph nodes. The functional immune modulators are proteins, cytokines, and metabolites, while the immune cells comprise myeloid cell lineages (neutrophils, monocytes, megakaryocytes, platelets, basophils, eosinophils) and lymphoid cell lineages (T cells, B cells/plasma cells, and NK cells).
Relationship and Interactions between TIME and STIE
There are extensive interactions between tumour immune microenvironment (TIME) and systemic tumour immune environment (STIE). Investigation on the TIME of tumour in situ and metastatic sites, along with STIE in blood, plays a significant role to understand the mechanism of tumour progression and overall cancer treatment (Xu et al., 2022). Looking for the key factors in STIE and TIME may identify pathway to improve the treatment efficiency of PD-1 inhibitors. As more clinical trials continue, effective combination of radiotherapy and immunotherapy will continue to make great breakthroughs.
Multiple Strategies to Prevent Immune Resistance
Multiple strategies targeting the TME have been investigated to prevent resistance to radiotherapy, chemotherapy, and immunotherapy (Wu et al., 2021 and references therein).
- Cancer-Associated Fibroblasts (CAFs) is the most abundant cell type in the TME that have the critical role in therapeutic resistance of tumour cells. Therefore, targeting CAF functions is a promising approach for tumour therapy.
- It has been shown to be an efficient tumour therapy strategy to stop the communication between tumour cells and their environment by targeting adhesion molecules, proteolytic enzymes, and ECM components.
- Different strategies can be used to target the immune system in TME and tackle cancers, because the immune system in TME influences the response of tumours to various clinical therapies.
- The low oxygen pressure and acidosis conditions in the TME affect a tumour’s response to treatment. Therefore, manipulating hypoxia and acidosis conditions in the TME can stop tumour progression.
- Capturing and leading the TME to increase drug delivery can significantly increase the efficacy of chemotherapeutic drugs.
Recommendations
Although manipulating the TME has shown success to overcome resistance to cancer/tumour treatments, most of these studies used the resistant cells in vitro (model study) (Wu et al., 2021 and references therein).
- Further research is needed in a real TME to understand the mechanisms underlying adaptive and non-adaptive resistance.
- The factors involved in the real TME might be crucial for acquired resistance in vivo.
- Simulate a real TME consisting of tumour cells and other types of cells and tumour-microenvironment-on-a-chip (TMOC) models can only partially mimic the real TME.
- An appropriate mouse models, or human organoids isolated from patient biopsies can be used to fully understand the role of TME.
- Monitoring the factors controlling the therapeutic effects of targeting the TME can improve the success of different therapies and clinical outcomes.
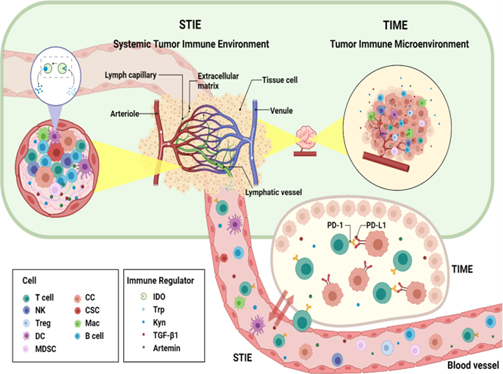
Reference
Kim T.K., Vandsemb E.N., Herbst R.S. et al., 2022. Adaptive immune resistance at the tumour site: mechanisms and therapeutic opportunities. Nat Rev Drug Discov 21, 529–540.
Spurrell E.L, Lockley M., 2014. Adaptive immunity in cancer immunology and therapeutics. ecancer https://doi.org/10.3332/ecancer.2014.441
Wu P, Gao W, Su M, Nice EC, Zhang W, Lin J, Xie N, 2021. Adaptive Mechanisms of Tumor Therapy Resistance Driven by Tumor Microenvironment. Front. Cell Dev. Biol. 9:641469.
Xu, L., Zou, C., Zhang, S. et al., 2022. Reshaping the systemic tumor immune environment (STIE) and tumor immune microenvironment (TIME) to enhance immunotherapy efficacy in solid tumors. J Hematol Oncol 15, 87. https://doi.org/10.1186/s13045-022-01307-2.